Categories of dust grains

 | Carbon rich dust grains
 | Amorphous Carbon (AC) (back to top)
 | Amorphous silicate was found in the disk of a bipolar PN, the Ant
nebula, Hen 2-154. It means the disk is still young.
(Chesneau et al., 2007A&A...473L..29C) |
|
 | SiC (back to top)
 | sub-classes: |
 | alpha-SiC: hexagonal and
rhomboedric types, very stable up to a temperature of 2700 deg. |
 | beta-SiC: cubic typs. It's more
stable than alpha-SiC above a temperature of 1600 deg. |
 | The two classes of SiC are similar in structure and
thermaldynamical property, can be
differentiated only through crystallography and IR spectrum. |
 | SiC feature in IRAS LWS spectra can be divided into 3 classes (a), (b) and (c), with each class
characterized by the width of 11.3um feature
and the presence (or no) of secondary features at ~ 8.6um, ~11.7um and ~ 12.8um. With the
secondary features subtracted, class(a) matches purest and smallest
grains of alpha-SiC, while class (c) matches polluted, coarse grains
of alpha-SiC. 24 M type stars are
associated with class (c) SiC feature: 00186+5940,
01030-3157, 01150+5732, 02169+5645, 05027-2158, 05208-0436,
05374+3153, 08555+1102, 09057+1325, 11011-6651, 13244-5904,
15298+0348, 15361+2441, 15492+4837, 16521-2153, 17072+1844,
17398-4344, 18401+2854, 21581+5707, 23420+5618, 00245-0652,
20111-4708, 21321+0136, 21377-0200. Many optical spectral type S stars are associated with class (c). Secondary features might be
carbonaceous dust responsible for IR continuum excess. (from
Papoular, 1988A&A...204..138P)
(figures: class (a), (b), (c) with
LRS type 4n (1st rwo) and 1n (2nd row) respectively)
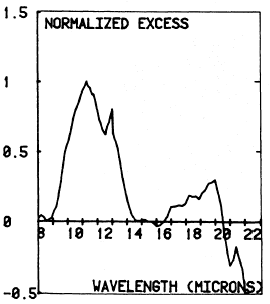
(figure: 6 sub- classes of class (a):
with different strength at 11.3um)
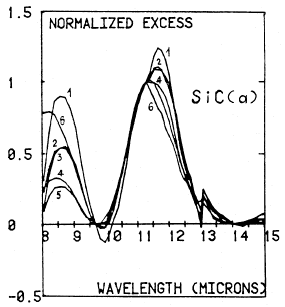 |
 | Laboratory measurement were made for beta-SiC grains with two
different shapes: one mainly
in small spherical shape (diameter =
27nm) and the other in ellipsoidal aggregates
(size of
100-500nm) of small crystallites (diameter = 15-20nm).
Laboratory spectral measurements
showed that, the spherical grains show double
peak features (at 11.0 and 12.1 um)
with the redder
peak stronger, while ellipsoidal aggregates show a single peak feature near 11.4um. The different
types of observed 11.3um (IRAS LRS) features can be explained by
linear combination of the
two types of grain shapes, but can not be interpreted in terms of
different polytypes. (from
Papoular et al., 1998A&A...329.1035P)
(figure: left is 6 types of observed
11.3um feature profiles--IRAS LRS; right is different combination of
the
two shapes: 1--ellipsoid; 2--0.78 ellipsoid + 0.39 sphere; 3--0.58
ellipsoid + 0.77 sphere.)
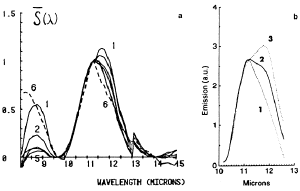 |
 | Alpha-SiC is stable up to 2700 deg. Beta-SiC
is also very stable but less thermodynamically
favored
than alpha-SiC. Beta-SiC is favored
when condensation takes place in vacuum.
Beta-SiC is more stable than alpha-SiC under 2000
deg. But beta-SiC will transform
into alpha-SiC when the temperature is higher than 2100
deg while the reverse reaction is very difficult.
Several important facts about SiC grains in
C-stars or ISM:
|
 | |
 | Carbon stars contribute roughly 1/3 of mass to ISM (Tielens, 1990). |
 | 11.3 um feature found in some C stars
which could be produced by SiC grains, e.g., Hackwell (1972),
Treffers and Cohen (1974); |
 | some optically thick C stars envelope
show very weak or no 11.3 um
feature. Whittet, Duley and Martin (1990MNRAS.244..427W). |
 | ISM extinction curve doesn't show 11.3um feature, where have
SiC grains from carbon stars gone? Stephens (1980),
Whittet, Duley and Martin (1990). |
 | Laboratory measurements showed that different alpha-SiC grain size distributions or impurities do not alter the width of the
11.3um feature, instead, they affect the position of the peak position. Larger
grains produce weaker 11.3um feature. Fiedemann et all. (1981).
They found a peak at 11.4 um in their experiment. |
 | Laboratory measurements showed that alpha-SiC
grains have a feature near 11.4 um,
while beta-SiC grains have a feature
near 11.0um. The feature positions of pure alpha- or beta-SiC
grains do not change with grain sizes. But alpha-SiC with 11% of impurities (carbon,
silicon, metallic ion, SiO2) does show a red
shift of emission peak with larger grain sizes. Borghesi
et al. (1985). |
 | small particles of a single shape produce several narrow
emission features between the longitudinal and transverse
vibrational wavelengths at 10.2um and 12.8um. Particles of different shapes would produce features
at different wavelengths between
these limits. A continuous distribution of shapes will produce
smooth feature with cut-on and cut-off wavelengths corresponding
to the longitudinal and transverse vibrational modes of SiC. (Treffers
and Cohen 1974, Kozasa et al., 1996) |
 | alpha-SiC is the best candidate to reproduce the strongest
11.3um features observed in IRAS LRS data, which means alpha-SiC is very similar only to the 11.3um
features observed in optically thin carbon stars whose
underlying continuum is the hotest. For cooler carbon stars, the
discrepancy becomes larger. |
 | Large size grains formed in the disk of Red Rectangle are
mostly beta-SiC. (Jura et al. 1997) |
 | AFGL 3068 was the first C stars
found to show 11.3um absorption
feature. (Jones et al., 1978) |
 | SiC feature is weaker in cooler carbon stars and companioned
by an emission feature around 8.5um
which may be attributed to alpha:C-H
on the surface of SiC grains. (Baron et al., 1987; Geobel et
al., 1995) |
 | Several facts about SiC grains in
meteorites:
|
 | |
 | SiC grains found in meteorites
are all beta-SiC (with 0.1-1.0 um
size. Bernatowicz et al., 1987, Geobel et al., 1995). Grains in
Murchison meteorite appear to be hexagonal (alpha-SiC) in
scanning electron microscope, but show Raman scpectroscopy
between 9.5-16.7um of beta-SiC. Hoppe et al., 1994. |
 | Meteorites are lack of SiC grains.
The reason is unknown yet. (Tang et al., 1989) |
 | Isotopic composition analysis indicated that SiC in meteorites may originate from four
different types of stars, including nova. (Tang et al.,
1989) |
 | From the analysis of C and N isotopic data, four distinct source of the two elements
in meteorites had been suggested (three of them are CNO cycle
(produce 13C, deplete 15N), He-burning
(produce 13C and 15N), and H-burning).
(Anders and Zinner, 1993) |
 | beta-SiC appear in large size grains in meteorites. (Anders
and Zinner, 1993) |
 | Given that it is geologically unlike to form SiC through
processing of solar nebula products in parent bodies of
meteorites, all beta-SiC grains in
meteorites should have a pre-solar origin. Because alpha-SiC is
difficult to transform into beta-SiC, the beta-SiC in meteorites
must be directly created . |
 | Ground based IR spectral observations discovered 4 carbon stars with SiC absorption feature
(including previously found AFGL 3068,
another three: IRAS 02408+5458, AFGL 2477, AFGL 5625).
All carbon stars with SiC emission or absorption features can be
best fitted by self-absorption model of alpha-SiC. The role of self-absorption may indicate that we don't
need coating of SiC grains with alpha:C-H to explain the diminution
of SiC in cooler stars. (from Speck et al., 1997MNRAS.288..431S) |
 | Majority of C stars were found to contain
mainly alpha-SiC grains, contrary to meteorites which contain
contain mainly beta-SiC. (from Speck,
et al., 2000IAUS..177..578S) |
 |
Laboratory measurement of the IR extinction curves
of mixtures of amorphous |
 |
carbon (AC) and SiC. (from Orofino et al., 1991A&A...252..315O)
(figure: a--AC with 25% alpha-SiC (by
mass, solid line) and 13% alpha-SiC (dashed line); b--AC with 25%
beta-SiC (solid line) and 10% beta-SiC (dashed line))
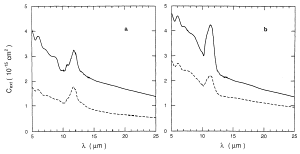
(figure: a--AC with 25% alpha-SiC
in KBr (solid line) and in vacuum (dashed line); b--AC with 25%
beta-SiC in KBr (solid line) and in vacuum (dashed line))
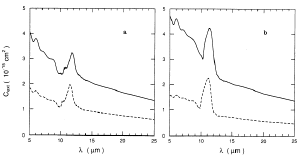 |
 |
Radiative transfer model fitting of C-stars showed
that, beside the continuum emission from
amorphous carbon dust grains, alpha-SiC
grains are needed for most of the sources to
reproduce the 11.3um feature, while beta_SiC is also occasionally needed for some
sources.
The presence of SiC grains is not correlated with optical thickness or any
other physical or
geometrical properties of the circumstellar envelope. However, the strength of the 11.3um
SiC feature is correlated with mass
loss rate (confirmed old results). (from Blanco et al., 1998A&A...330..505B)
(Here is an example of the RT model fit of IRAS 06331+3829. Dots are
data, line is model.)
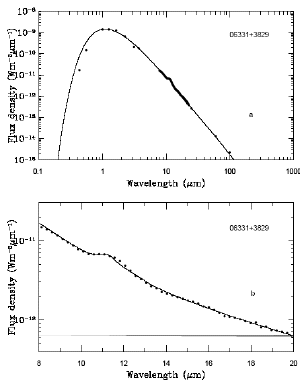 |
 | If SiC is the carrier of 21um feature, the 21um/11.3um strength ratio
requires too strong 21um
resonance that pure SiC can not supply. Large impurity of C
could be able to produce enhanced
21um. (need more laboratory work on this idea.) (from Jiang et al., 2005ApJ...630L..77J) |
|
 | PAHs
(back to top)
 | IR emission spectrum of a mixture of Amorphous
silicates and graphite grains and
varying amount of PAH particles. Only
one-photon heating is considered. They present updated
IR absorption properties of PAHs. They give a grid
of IR emission models with various compositions that agree to Spitzer
observations of the Milky Way galaxy. (from Draine & Li, 2007ApJ...657..810D)
(figure: left -- Absorption
cross sections of neutral and ionized PAH; right
--
absorption cross section of ionized carbonaceous grains)
|
|
|
 | Oxygen rich dust grains
|
 | Other issues
(back to top)
 | A radiative transfer code was used to fit the observed IR SED of two OH/IR
stars IRAS 17004-4119 and IRAS
17411-3154. They concluded that the 9.8 and
17.5um absorption features are due to amorphous silicates, the at 11.2um absorption shoulder and 33.6um
emission are due to crystalline forsterite, the
3.09um absoprtion and 43um emission
features are due to crystalline water ice, the 40.5um
emission is due to crystalline diopside (MgCaSi2O6), the 43um emission band is also due to crystalline
enstatite. The crystalline silicates represent 35%
and 25% of amorphous dust mass in the
above two stars respectively. About 10% of olivines and
65-100% of pyroxenes were found to be crystalline. (from Demyk et al., 2000A&A...364..170D)
(figure: mass absorption coefficients
of amorphous and crystalline silicates used in the paper. Upper panel -- solid line
is amorphous olivine, dashed line is amorphous pyroxene, dotted line is FeO,
dot-dashed line is crystalline water ice; Lower
panel -- solid line is crystalline
forsterite, dashed line is crystalline enstatite, dot-dashed line is
diopside.)
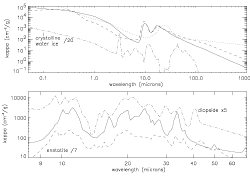 |
|
(back to top)
|